Dr Kenny Jolley
Lecturer – Chemistry
Dr Kenny Jolley’s expertise is in theoretical chemistry. He has more than 15 years’ experience in a wide range of computational modelling techniques from density functional theory (DFT) calculations to force-field based methods using Molecular Dynamics (MD). His current work explores how nuclear graphite responds to the high dose irradiation it receives in a nuclear reactor. Expanding our understanding of this material and its behaviour will enhance the safety and longevity of nuclear power stations.
Enhancing the safety and longevity of nuclear power stations
Nuclear graphite is used as a building material and neutron moderator within the reactor cores of the UK’s Advanced Gas-cooled Reactors (AGRs) – and will likely be used in the next generation of facilities. By deepening our knowledge of nuclear graphite, Dr Jolley is helping to improve the resilience and performance of current and future nuclear energy generation – supporting the transition from fossil fuels as we race to reduce our carbon footprint.
About 20% of the UK’s electricity is produced by nuclear power, but almost half of the current capacity is due to be retired by 2025.
Just as these older reactors are being decommissioned, there is increasing demand for electricity – particularly from renewable and low carbon sources – as more of us adopt electric vehicles and move to electric, rather than gas, heating and cooling.
The main reason nuclear reactors close is the presence of an unsafe number of cracks in the nuclear graphite bricks in the reactor core – it’s why Hunterston B was shut down in January 2022.
The high levels of radiation within a reactor core damage the graphite bricks, causing a range of problems including dimensional change, creep, radiolytic oxidation and cracking.
A cracked brick could crumble into the rod channels, blocking them – preventing fuel rods from being removed or control rods from being inserted – potentially leading to a dangerous situation where the nuclear reaction can’t be controlled.
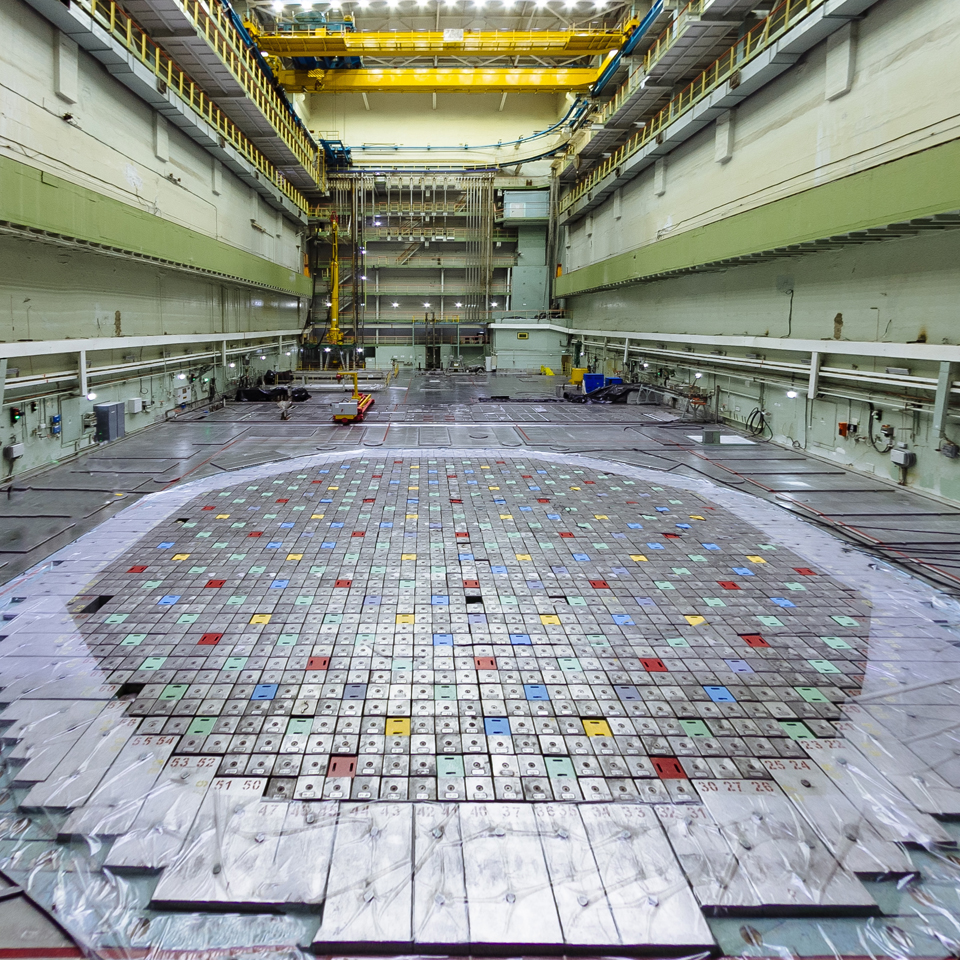
It’s not possible to replace cracked or damaged bricks individually. Introducing a new brick could produce further strains in the structure causing additional cracks. What’s more, this kind of activity would pose a risk to workers handling radioactive materials.
By deepening our understanding of nuclear graphite and how it behaves within the hostile environment of a reactor, we hope to safely extend the runtime of existing AGRs and design new materials that will improve the resilience and performance of next generation reactors.
My current research – funded by a New Investigator Award from the Engineering and Physical Sciences Research Council – is investigating how nuclear graphite’s microstructure affects the properties and integrity of graphite bricks in high temperature and high irradiation conditions.
My aim is to be able to predict when a brick with a given microstructure will fail. This will provide the industry with data about the safety of current reactors as well as a template for resilient graphite materials for use in Generation IV nuclear technologies.
I’m also part of the SlowCat (Securing a Sustainable Future using Low Dimensional Catalysts) project that aims to find energy efficient ways to generate biofuels as a promising alternative to declining fossil fuels from waste materials.
It is important to me that my research has real-world applications and is helping to address the challenge of developing clean and safe alternatives to fossil fuels.
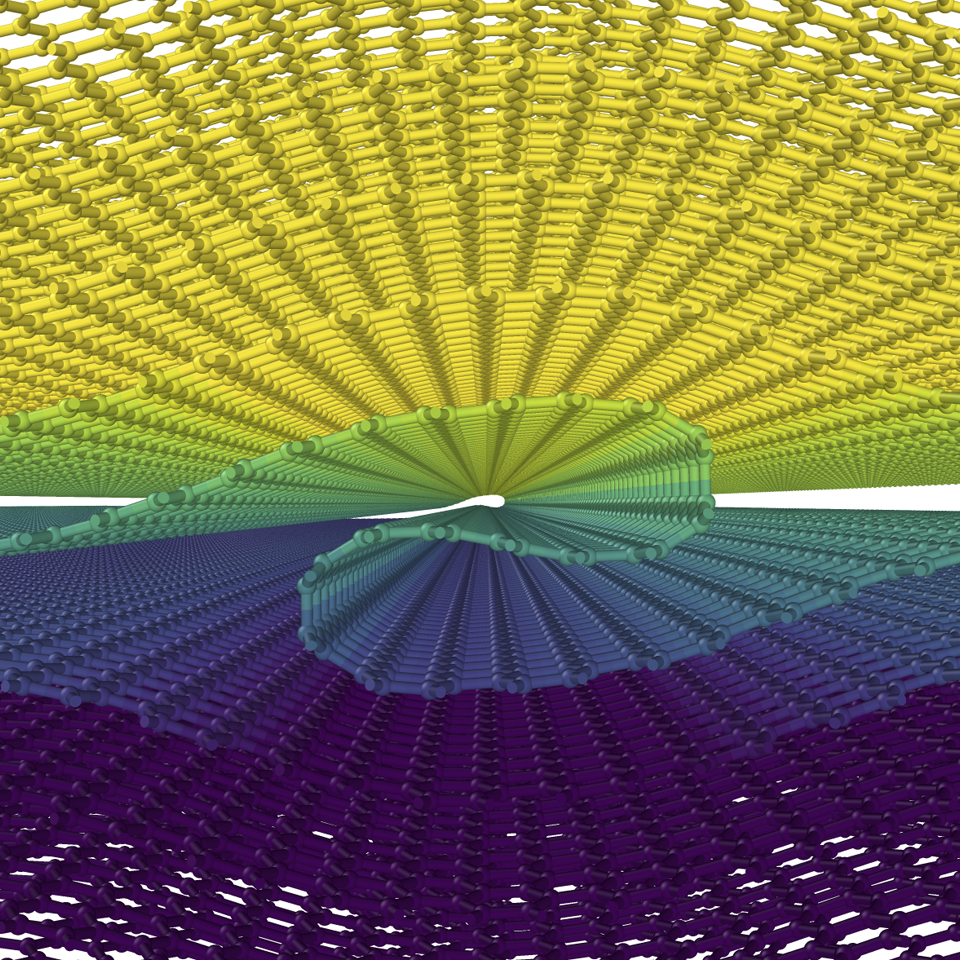
Currently, their production is a highly energy-intensive process. Catalysts such as platinum can speed up reactions and reduce the energy requirements. However, we want to use as little of this rare and expensive element as possible.
Platinum nanoclusters – or even single platinum atoms – distributed on a 2D surface have the highest theoretical active surface area possible. So, we’re synthesising platinum nanoparticles and distributing them on graphene oxide surfaces for use as catalysts.
Using experimental and theoretical techniques, we’re investigating the stability of these nanoclusters under reaction conditions – we don't want them all dancing around or clumping together – and how effective they are as a catalyst for biofuel production.
It's good to know that my work is contributing to the research and development of low-carbon energy technologies – helping us to reduce emissions while meeting the ever-growing demand for sustainable electricity.
My research journey
Physics and computing were my favourite subjects at school – I taught myself how to code and was fascinated by the theoretical and electronic elements of physics – and I’ve always enjoyed exploring how things work and why.
As I’ve progressed through my studies and research, my work has become increasingly interdisciplinary – the boundaries between maths, physics and chemistry are really blurred. You need maths to understand physical laws and chemistry to understand materials.
I completed my undergraduate and postgraduate studies in Physics at the University of Leicester. My PhD thesis developed and applied multi-scale computer simulation methods for nano-engineering applications, resulting in several publications.
After my PhD, I moved to The University of Nottingham’s Mathematical Sciences Department to work with Professor Richard Graham. During my three years as a Postdoctoral Researcher, I helped to develop new mathematical models and techniques for simulating flow-induced nucleation in polymers. Our fast nucleation algorithm underpins ongoing research in this area.
In 2013, I moved to Loughborough to join Professor Roger Smith’s research group, working on simulations of nuclear waste glasses. Our work demonstrated that amorphous glass structures can reconstruct to similar stable amorphous structures on very short timescales, giving the glass its high resistance to radiation damage.
My next project – “Understanding and Improvement of Graphite for Nuclear Fission” (UNIGRAF) – was with Professor Roger Smith and Dr Houzheng Wu. Building on Dr Benjamin März and Dr Wu’s experimental observations, we focused on understanding the mesoscale structures that form during the preparation and irradiation of nuclear graphite.
I secured my current role as Lecturer in October 2017, and since 2019 have led the research group investigating how atomistic defects – such as basal dislocations – affect the properties and dimensional change of graphite.
Over the years, I have established positive partnerships with a number of other researchers worldwide as well as industry collaborators, including Sinosteel and EDF. My lectureship position is part-funded by EDF, and we meet regularly – either on campus or at their facilities – to discuss our ongoing research.
It is important to me that my research has real-world applications and is helping to address the challenge of developing sustainable and safe alternatives to fossil fuels.